An Overview of Third Generation Solar Cells: Definition, Structure, Types & Efficiency
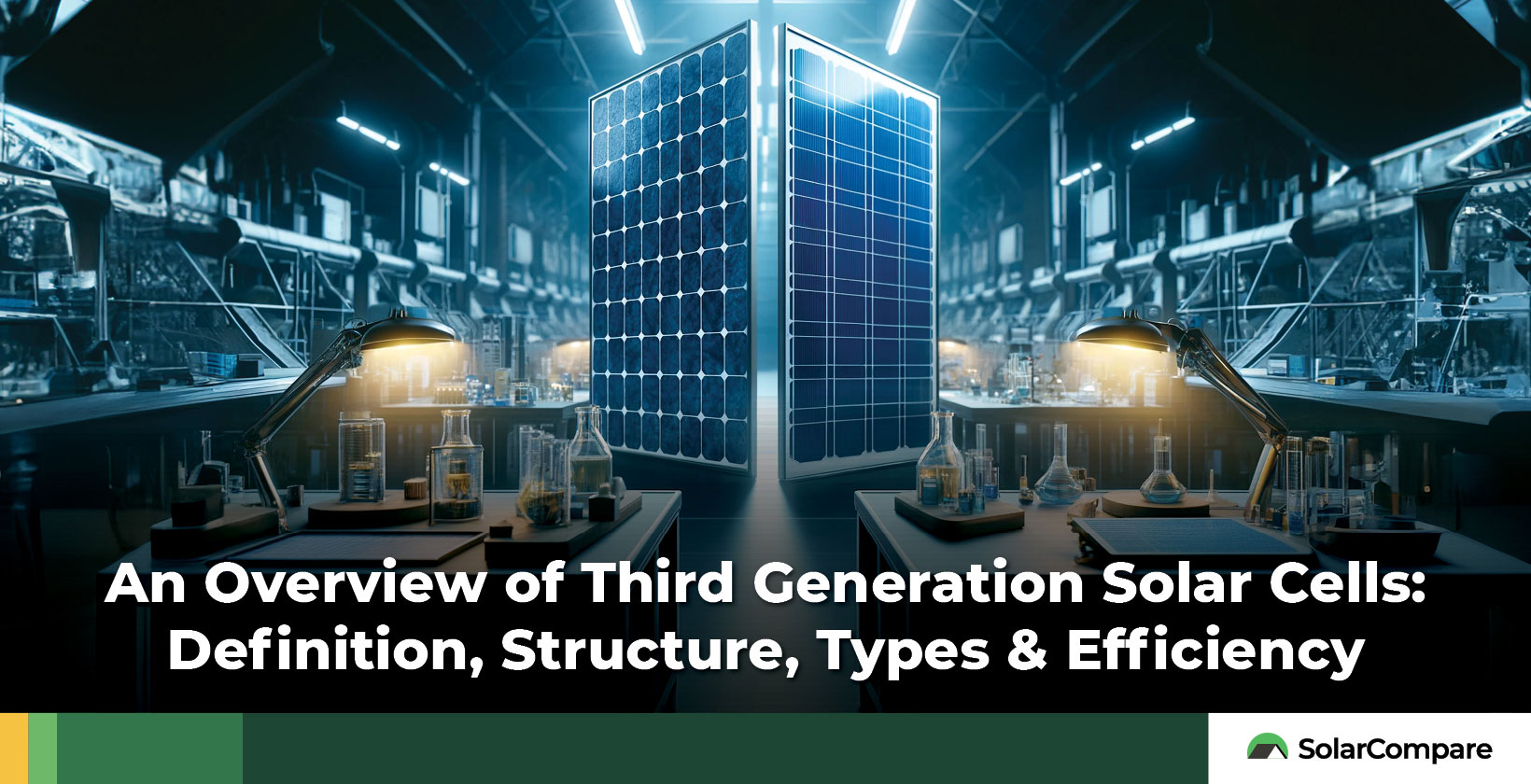
A third generation solar cell is an advanced photovoltaic (PV) device designed to overcome the limitations of first and second generation cells. These cells aim for higher efficiencies using modern chemicals and technologies while minimizing manufacturing costs. The primary goal of third generation solar cells is efficient, affordable sunlight-to-electricity conversion. They aim to surpass the Shockley-Queisser limit, which limits single-layer solar cell efficiency to about 33.7%, by using tandem structures and modern chemicals to break this efficiency barrier. Recent advancements have made some third gen solar cells easier and more stable to produce, achieving nearly 25% efficiency and longer lifespans—a significant step towards commercial use.
Key third-generation solar cell materials include perovskite (PSCs), dye-sensitized (DSSCs), copper zinc tin sulfide (CZTS), and quantum dot solar cells. Perovskite cells are notable for their high efficiency and simple processing. Dye-sensitized cells are versatile and cost-effective, ideal for lightweight applications. CZTS cells use abundant, non-toxic materials, offering an eco-friendly alternative. Meanwhile, quantum dot cells employ nano-sized semiconductors to boost light absorption and electricity generation, targeting unmatched efficiency levels. These materials are crucial for developing sustainable, affordable solar energy solutions.
Different third-generation cells are designed to meet a variety of needs. Organic cells, known for their flexibility, are perfect for wearable technology. Perovskite cells, on the other hand, combine high efficiency with low production costs, making them ideal for large-scale energy production. Lastly, quantum dot cells allow for adjustable efficiency, making them adaptable to various environments.
Third-generation solar cells use semiconductor electrodes, dyes, electrolytes, surfactants, and counter electrodes, going beyond silicon to embrace various semiconductor technologies. This variety leads to higher efficiencies and better solar energy capture, significantly advancing solar power. Innovations in nanotechnology and materials such as gallium arsenide in multi-junction cells, which capture a wider range of the solar spectrum, have reached efficiencies up to 44.7%.
Slowly making their way into the market, third-generation solar cells promise future solar technology with flexibility, potential cost savings, and higher efficiency. Perovskite solar cells (PSCs) are particularly promising for urban power generation, seamlessly integrating into building exteriors like roofs and façades with their transparency, color options, and high energy output.
The evolution of solar cell technology across three generations reveals significant progress and challenges, pointing towards a sustainable energy future. Despite durability concerns, the potential impact of third-generation solar cells on the industry is immense, underlining the importance of ongoing innovation.
What is a Third Generation Solar Cell?
A third generation solar cell is an advanced type of photovoltaic (PV) device designed to overcome the limitations of first and second-generation cells. Third-generation solar cells aim to achieve higher efficiencies through the use of modern chemical compounds and innovative technologies while maintaining low manufacturing costs. Among the most prominent materials leveraged in third generation solar cells are perovskite solar cells, dye-sensitized solar cells, copper zinc tin sulphide (CZTS) solar cells, and those based on quantum dot technology. Perovskite solar cells are celebrated for their high efficiency and easy processing, making them a frontrunner in the race towards commercial viability. Dye-sensitized solar cells offer versatility and lower costs and are suitable for less intensive applications, such as electronic devices, and portable power systems. CZTS cells stand out for their use of abundant, non-toxic materials, presenting a more environmentally friendly option. Quantum dot solar cells, meanwhile, utilize nano-sized semiconductors to improve light absorption and electricity generation, heralding a future of solar cells with potentially unprecedented efficiency levels. Together, these materials form the backbone of third generation solar cells, pushing the envelope in the quest for sustainable, cost-effective solar energy solutions.
Perovskite solar cells are advancing third-generation solar technology, potentially exceeding the efficiency of conventional silicon panels at a reduced cost. Recent studies highlight a breakthrough method that allows Perovskite cells to be manufactured more easily and with greater stability, using an innovative additive. This advancement not only increases the potential efficiency of perovskite cells to nearly 25%, but also improves their longevity, marking a significant step towards commercializing a technology that could revolutionize solar power by enhancing efficiency and reducing production costs.
What is the job of a Third Generation Solar Cell?
The primary job of third generation solar cells, like all solar cells, is to convert sunlight into electricity at a high efficiency while maintaining low production costs. They achieve this by absorbing photons (sunlight particles) to generate charge carriers (electrons and holes) in a semiconductor material, which then flow through an external circuit to perform electrical work. However, third-generation solar cells are designed to exceed the Shockley-Queisser limit, a theoretical threshold that sets the maximum efficiency at approximately 33.7% for single-layer solar cells in specific scenarios. Third-generation solar cells achieve higher efficiency levels by employing innovative techniques such as tandem structures, and modern chemical compounds, allowing them to surpass the efficiency constraints predicted by the Shockley-Queisser limit.
Different types of third-generation solar cells are being developed to address specific challenges and cater to various needs. For example, organic solar cells are flexible and lightweight, making them suitable for applications in wearable technology and portable electronics. Perovskite solar cells offer high efficiency and can be manufactured using low-cost processes, making them ideal for large-scale solar energy generation. Quantum dot solar cells have the potential for tunable bandgaps, allowing for more efficient energy conversion and integration into diverse environments.
High efficiency is a crucial goal in solar technology for several reasons. Firstly, it maximizes the electricity output from a given amount of sunlight, making solar energy more cost-effective and competitive with other forms of energy generation. Secondly, higher efficiency means that fewer solar panels are needed to achieve the desired power output, reducing installation space and overall costs. Lastly, improved efficiency contributes to the sustainability of solar energy by enhancing its environmental benefits and reducing its carbon footprint.
What is the Structure of a Third Generation Solar Cell?
Third Generation solar cells utilise two main types of solar cell structure to improve solar panel efficiency by increasing the amount of light absorbed at different wavelengths. The most common type of structure used by third-generation solar cells utilizes a multi-layer (tandem) structure in which multiple layers of thin-film silicon cells are stacked to create a ‘multi-junction’ cell that is capable of absorbing light at different bandwidths. Whereas more advanced, theoretical, or experimental third generation cells are looking to use 'frequency conversion' technology. Frequency conversion technology is an advanced and largely theoretical solar cell structure that makes use of rare earth ions to change the frequency of photons closer to the band gap of solar cell semiconductors.
What are the Main Types of Third Generation Solar Cells?
There are three main types of third generation solar cells. These are Dye-sensitized solar cells (DSSCs), Quantum dot-sensitized solar cells (QDSSCs) and Perovskite-sensitized solar cells (PSCs).
More information on each third-generation solar cell type is given below:
- Dye-sensitized solar cells (DSSCs): DSSCs use a dye to absorb sunlight and release electrons, attached to semiconductor particles like titanium dioxide. They are known for their relatively good efficiency, simple manufacturing process, and lower costs compared to silicon-based cells.
- Quantum dot-sensitized solar cells (QDSSCs): QDSSCs use nanoparticles to generate multiple electron-hole pairs from a single photon, potentially surpassing traditional cell efficiency.
- Perovskite-sensitized solar cells (PSCs): PSCs use perovskite compounds for light harvesting and charge transport, offering high-efficiency rates and requiring thinner films compared to silicon cells.
Each of the three main types of third-generation solar cells uses unique technologies to improve upon the limitations of previous generations. Additionally, each of these types leverages unique material properties to enhance light absorption, reduce cost, and improve the overall efficiency of converting solar energy into electricity.
1. Dye-sensitized Solar Cells(DSSCs)
Dye-sensitized solar cells (DSSCs) utilize a photosensitive dye to absorb sunlight and release electrons. This dye is attached to a layer of semiconductor particles, such as titanium dioxide, over a conductive substrate which transports the electrons.
Dye-sensitized solar cells (DSSCs) are notable for their relatively good efficiency, ease of manufacturing, and lower production costs compared to traditional silicon-based cells. With efficiencies typically reaching up to 12%, dye-sensitized solar cells (DSSCs) are less efficient than some other third-generation solar cells. However, their ability to function under low light conditions and their flexibility in terms of aesthetics (as they are made semi-transparent or colored) make them attractive for building-integrated photovoltaics (BIPV) and consumer electronics.
Compared to other solar cell types, DSSCs use lower-cost materials and have a simpler manufacturing process, reducing overall costs. However, they may have a shorter lifespan and face challenges with dye and electrolyte stability under extended sunlight exposure. In contrast, perovskite and quantum dot solar cells utilize advanced materials for potentially higher efficiencies and longer lifetimes, but with higher manufacturing complexities and costs.
2. Quantum dot-sensitized Solar Cells (QDSSCs)
Quantum dot-sensitized solar cells (QDSSCs) use nanoparticles that generate multiple electron-hole pairs from a single photon, potentially surpassing the efficiency of traditional solar cells. This is due to the unique attribute of quantum dot nanoparticles known as the quantum confinement effect, allowing them to absorb a wider spectrum of solar radiation and contribute to higher photoelectric conversion efficiency.
The tunable bandgap of QDSSCs allows for customization of the wavelengths of light absorbed, making them adaptable to different environments. However, challenges such as long-term stability and complex production processes need to be addressed for commercial viability. Further research is required to overcome issues such as the toxicity of some quantum dot materials and the cells' durability in real-world conditions.
3. Perovskite-sensitized Solar Cells (PSCs)
Perovskite solar cells (PSCs) have attracted much attention due to their rapidly increasing efficiency rates. These cells use a perovskite-structured compound as the active layer for light harvesting and charge transport.
Perovskite-sensitized solar cells use perovskite compounds in the active layer, known for high light absorption and long charge-carrier diffusion lengths. Unlike silicon-based cells, they require thinner films for comparable absorption, reducing material usage and potential manufacturing costs. Perovskite cells are usually processed using simple methods like solution processing, similar to printing. These cells have achieved impressive power conversion efficiency, surpassing traditional silicon cells. However, their stability and lead content remain concerns, driving research for encapsulation techniques and lead-free alternatives. Despite challenges, perovskite solar cells offer high efficiency and low-cost potential, making them a leading third-generation solar technology.
The commonly used semiconductors in third-generation solar cells include amorphous silicon (a-Si) and gallium arsenide (GaAs). These materials have been extensively researched and used in various solar cell technologies, including thin-film and multi-junction cells. However, with the recent advancements in third-generation solar technology, newer semiconductors such as quantum dots and perovskites are gaining attention for their potential to overcome the limitations of traditional silicon-based cells, including high production costs and limited efficiency.
What is the Maximum Efficiency of a Third Generation Solar Cell?
The maximum efficiency achieved by third-generation solar cells in laboratory settings has been climbing steadily, with some perovskite solar cells reaching efficiencies above 25%. As research continues, there's potential for this efficiency to rise even further. Traditionally, silicon cells often suffer from high production costs due to the materials and processes involved, such as the need for high-purity silicon and energy-intensive manufacturing.
Additionally, traditional solar cells are limited by the Shockley-Queisser limit, which caps the maximum theoretical efficiency of a silicon solar cell at around 30%. Another challenge is solar cell rigidity. Silicon cells are typically encased in glass, making them unsuitable for applications requiring flexibility. Third-generation solar cells, including perovskites and quantum dots, promise higher efficiencies beyond the traditional limit and offer a path to more versatile and less expensive solar applications.
What is the Commonly used Semiconductor in a Third Generation Solar Cell?
Commonly used semiconductors in third-generation solar cells include gallium arsenide, amorphous silicon, and Metal-halide perovskites. More information on each semiconductor used in these third-generation cells is given below.
- Gallium Arsenide (GaAs): GaAs is a compound of the elements gallium and arsenic. It is used in high-efficiency 3rd generation solar cells due to its ability to absorb sunlight well.
- Amorphous Silicon (a-Si): Amorphous silicon is a non-crystalline form of silicon. It is commonly used in thin-film solar cells and has the advantage of being flexible and lightweight.
- Metal-Halide Perovskites (Perovskite): Perovskite refers to a class of materials with a specific crystal structure. Metal-halide perovskites have emerged as a promising material for third-generation solar cells due to their high efficiency and low cost.
Third-generation solar cells utilize semiconductor electrodes, dyes, electrolytes, surfactants, and counter electrodes as key components. Third-generation solar technologies are diversifying the materials and methods used in photovoltaic cells, moving beyond traditional silicon to include a variety of semiconductor technologies. These innovations offer the promise of higher efficiencies and the ability to harness solar energy more effectively, marking a significant advancement in the field of solar power.
Third-generation solar technologies explore various material methods, including nanotechnology, to enhance efficiency and functionality. Semiconductors are at the core of this innovation, with a focus on silicon nanostructures. Researchers manipulate silicon at the nanoscale to overcome limitations set by the Shockley–Queisser limit, which is a theoretic limit that caps the efficiency of solar cells at around 30-32%. Nanoscale technologies such as aluminum nanocylinders are able to enhance the efficiency of silicon solar cells. Introducing materials like gallium arsenide in multi-junction cells broadens the spectrum of captured solar energy. By stacking layers of materials with different bandgaps, these cells capture photons across a wider range of energies, significantly boosting efficiency, with GaAs cells achieving efficiencies of up to 44.7%.
How much is the average cost of a Third Generation Solar Cell?
The average cost of a third generation solar cell is difficult to determine as this technology is not readily available on the market. Third generation PV cells are in continuous development, still being optimized for higher efficiency and lower production costs. Solar energy technicians are working towards a breakthrough that could significantly reduce solar energy expenses, projecting that perovskite solar cells might eventually cost as low as $0.10 per watt. This is markedly lower compared to current crystalline silicon solar cell costs, which cost around $0.30 per cell. Such advancements could transform the solar power industry, making sustainable energy more affordable and accessible on a global scale.
Are Third Generation Solar Cells installed in modern Solar Panels?
No, third-generation solar cells are not yet commonly installed in modern solar panels. Third-generation cell technologies like dye-sensitized solar cells (DSSCs) are being gradually integrated into the market and hold considerable promise for future solar applications due to their advantages in terms of flexibility, potential costs, and efficiency. Perovskite solar cells (PSCs) hold immense promise for revolutionizing power generation, particularly in urban landscapes where the seamless integration of photovoltaic technology is paramount. Their exceptional transparency, diverse color options, and impressive energy output allow PSCs to merge harmoniously with building exteriors such as roofs and facades. Perovskite solar cells (PSCs) excel at reaching niches beyond conventional silicon cells, thereby expanding the choice of renewable energy applications. PSCs find utility in various sectors, such as portable electronics, building-integrated photovoltaics, smart windows, and even powering satellites in space. The versatility and efficiency of perovskite cells make them a promising technology for the future of solar panel technology. Despite facing certain obstacles, by 2026, all major silicon manufacturers will have pledged to include perovskite products. Revolutionizing power generation with the seamless integration of photovoltaic technology in urban landscapes.
Are Third Generation Solar Cells the same as Photovoltaic Cells?
Yes, third generation solar cells are a type of photovoltaic (PV) cell, which is any cell that directly converts light into electricity. However, not all PV cells are third generation; this term specifically refers to a new class of non-traditional solar technologies. First- and second-generation PV cells are the commonly known silicon wafer-based or thin-film cells, while third generation encompasses various emerging technologies such as organic solar cells, dye-sensitized solar cells, and perovskite solar cells. These newer technologies offer different advantages over traditional silicon solar panels, such as increased efficiency and lower costs. Therefore, while they fall under the category of PV cells, third generation solar cells are a distinct and innovative approach to harnessing solar energy. With further research and development, they have the potential to revolutionize the field of renewable energy. So, while it is important to understand that third-generation solar cells are a type of photovoltaic cell, it is equally crucial to recognize their unique characteristics and potential impact on the renewable energy industry.
Are Third Generation solar cells consistent in producing solar energy?
Improvements are being made to enhance the consistency of solar energy production in third-generation solar cells. The stability of materials used in third-generation solar cells plays a crucial role in ensuring consistent energy production. Perovskite cells, in particular, have shown some challenges in terms of long-term stability, although significant progress is being made in this area. However, endurance under different environmental conditions remains a significant obstacle to overcome. For example, the stability of third-generation photovoltaic modules in different working conditions, such as high humidity, high temperature, and continuous light illumination, is a major challenge that has yet to be overcome. Furthermore, operating stability issues continue to hinder the practical applications of third-generation solar cells. Therefore, advancements in third generation solar technology and cell design are crucial to improve their resilience and ensure reliable functionality over time, especially in the face of increasing climatic stressors.
What is the difference between Third Generation Solar Cells and Multi-Junction Solar Cells?
The main difference between multi-junction solar cells and third-generation solar cells relates to their distinct approaches to increasing efficiency. Multi-junction solar cells use multiple p-n junctions to capture a broader solar spectrum, while third-generation cells use more modern technologies to harness higher current output through multiple exciton generation.
Moreover, third-generation solar cells are not limited to crystalline materials like multi-junction cells. They are fabricated using a wide range of materials, such as organic polymers, perovskite compounds, and quantum dots. This flexibility in material choice offers potential advantages in terms of cost-effectiveness and scalability. The use of abundant and low-cost materials, combined with innovative manufacturing techniques, contributes to reducing production costs and making solar energy more accessible.
While both multi-junction solar cells and third-generation solar cells aim to increase efficiency and reduce production costs, they employ different strategies. Multi-junction solar cells optimize the absorption of different segments of the solar spectrum through multiple p-n junctions, while third-generation solar cells leverage alternative principles like multiple exciton generation and diverse material options. These distinct approaches contribute to the advancement of solar cell technology and the realization of more efficient and cost-effective solar energy systems.
What differentiates Third Generation Solar Cells from other Generations?
Third-generation solar cells stand out from earlier generations by utilizing non-traditional materials and technologies to overcome efficiency limitations. While first and second-generation cells mainly rely on pure silicon and thin-film technologies, third-generation cells incorporate multiple materials with complementary properties. This characteristic allows them to achieve higher efficiencies and potentially lower costs. Additionally, third-generation solar cells offer increased flexibility and versatility in their applications.
However, it's important to note that third-generation solar cells, such as perovskite solar cells (PSCs), have not yet matched the proven longevity of traditional crystalline silicon technology, which usually operates reliably for over 30 years. The highest reported stability for PSCs is currently around 10,000 hours, slightly over a year. This disparity highlights the ongoing need for research and development to enhance the durability of emerging technologies and meet or exceed the industry-standard lifespan set by their predecessors.
The evolution across three generations of solar cell technology reveals clear progress. Each era has introduced distinct advancements and hurdles, thus shaping the sustainable energy landscape. Despite the current disparity in longevity, the potential of third-generation solar cells to revolutionize the industry is promising, driving the necessity for continuous innovation and improvement.