Thin-Film Solar Cells: Definition, Types & Costs
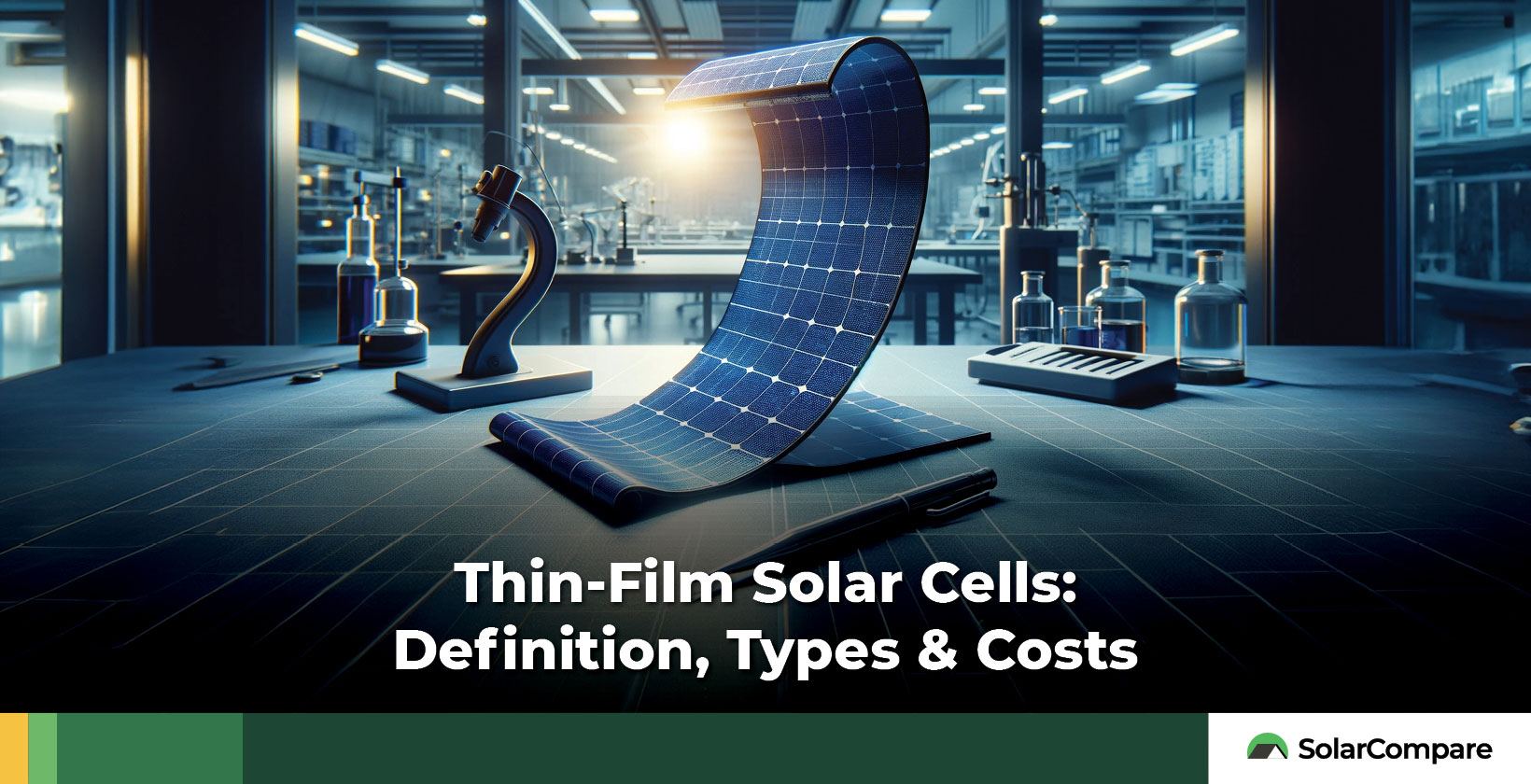
Thin-film solar cells are a type of photovoltaic device that converts sunlight into electricity using layers of semiconductor materials applied thinly over a flexible substrate. Thin-film cells are valued for their flexibility, allowing installation on diverse surfaces. They are cost-effective, due to reduced material use and simple production processes. The adaptability of thin-film solar panels makes them ideal for applications where conventional panels cannot be used, including curved architectural elements, vehicles such as RVs and boats, and temporary or mobile setups.
Thin-film solar cells typically have a lower efficiency of 7-22%, compared to traditional monocrystalline and polycrystalline types. The thin photovoltaic layers of thin-film cells limit their sunlight absorption and electricity generation capabilities, although this same characteristic grants them greater flexibility.
There are four main types of thin-film solar cells, each distinguished by unique materials and characteristics. Amorphous Silicon (a-Si) solar cells are notable for their flexibility and cost-effectiveness, despite lower efficiency and vulnerability to light-induced degradation. Cadmium Telluride (CdTe) cells, which utilize a cadmium and tellurium compound, offer simplicity in manufacturing and effective sunlight absorption but raise health and environmental concerns due to the toxicity of cadmium. Copper Indium Gallium Selenide (CIGS) solar cells, known for their low environmental impact and adaptability to diffuse light, face challenges related to water vapor sensitivity, which affects their long-term durability. Gallium Arsenide (GaAs) solar cells are a specialized type of photovoltaic technology known for exceptional semiconductor properties.
There are several key advantages of thin-film panels, despite demonstrating lower efficiency compared to traditional panels. Thin-film solar cells are incredibly lightweight and flexible compared to traditional silicon-based solar panels. This makes them adaptable to a wider range of spaces and applications. Recent research at MIT has led to the development of thin-film cells that are so lightweight that they can be rested on a soap bubble.
Thin film solar cells typically cost $0.50 to $1 per watt on average, but they typically have a shorter lifespan of 10-20 years compared to other types of solar panels. In contrast, monocrystalline panels generally last 35 to 50 years, and polycrystalline panels around 25 to 35 years.
What are Thin-Film Solar Cells?
Thin-film solar cells are a type of solar panel or semiconductor devices that convert sunlight into electricity through the photovoltaic effect. Unlike traditional solar panels, which use thick wafers of crystalline silicon, thin-film cells are made of semiconductor layers that are only microns thick. This makes them much lighter and more flexible than traditional solar cells.
The concept of thin-film solar cells was first discovered in the 1970s by a pioneering team at the Institute of Energy Conversion at the University of Delaware, USA. The transition from theoretical design to practical application began in the 1980s, marked by key advancements that overcame earlier limitations in efficiency and manufacturing. These improvements made thin-film solar cells viable for small-scale applications such as powering calculators and watches. Entering the 21st century, the evolution of thin-film solar technology accelerated, bridging the gap from small-scale gadgets to larger energy generation systems. Advancements in manufacturing techniques and efficiency gains have expanded their use to more demanding applications, including commercial rooftop installations, and even large-scale solar farms.
Thin-film solar cells come in various types, each with unique materials and characteristics. The main thin-film solar panel types include Amorphous Silicon (a-Si) Solar Cells, known for their flexibility and low-light performance; Cadmium Telluride (CdTe) Solar Cells, recognized for their simplicity in manufacturing and effective sunlight absorption; Copper Indium Gallium Selenide (CIGS) Solar Cells, notable for their environmental friendliness and efficiency in diffuse light environments; and Gallium Arsenide (GaAs) Solar Cells, distinguished by their high-temperature resistance and exceptional efficiency rates.
Thin-film solar cells are produced through the deposition of one or more thin layers (referred to as thin films or TFs) of photovoltaic material onto a substrate. The most common substrates are glass, plastic, or metal on which thin layers of either amorphous silicon (a-Si), cadmium telluride (CdTe), copper indium gallium selenide (CIGS), or gallium arsenide (GaAs) are deposited.
The two main advantages of thin-film solar cells are flexibility and cost-effectiveness. The flexibility arises from their unique construction, where photovoltaic materials are applied in extremely thin layers, often less than a few micrometers thick. This ultra-thin profile allows thin-film cells to be flexible and lightweight, making them ideal for a wide range of surfaces, including curved and irregular shapes, where traditional rigid panels are impractical.
The cost-effectiveness of thin-film solar cells stems primarily from their lower material usage and simpler manufacturing process compared to traditional crystalline silicon solar cells. Since they require less material, the production costs are reduced, making the overall cost of solar installations cheaper. This combination of flexibility and cost-efficiency makes thin-film solar cells an attractive option for both large-scale and specialized applications. Additionally, thin-film solar cells provide an aesthetic benefit. Leading manufacturers offer frameless, glass-on-glass thin-film models, which are attractive for homeowners prioritizing aesthetics for their PV installation.
How do Thin-film Solar Cells work?
Thin-film solar cells operate on the principle of the photovoltaic effect, where sunlight is converted into electricity. These cells are made up of thin layers of photon-absorbing materials (materials able to capture light particles), such as amorphous silicon or cadmium telluride, applied onto a flexible substrate. When sunlight strikes these layers, it excites electrons, creating electron-hole pairs. Then, as electrons move towards one electrode and holes towards the other, an electric current forms. When channeled through an external circuit, the current produces electricity.
Unlike traditional solar cells, which need thicker layers of their photovoltaic material to function effectively, thin-film solar cells achieve the same functionality with much thinner layers. The reduction in material usage makes thin-film solar cells more cost-effective and environmentally friendly, as they consume less of the resources (like silicon, cadmium, or telluride) needed for their production. However, this advantage comes with a trade-off in efficiency; thin-film solar cells generally have a lower capacity to convert sunlight into electricity compared to their thicker, traditional counterparts.
How Efficient Are Thin-Film Solar Cells?
Thin-film solar cells generally exhibit lower efficiency and power capacity compared to monocrystalline and polycrystalline solar cell types. The efficiency of thin-film cells typically ranges from about 7% to 22%, depending on the photovoltaic materials used. In contrast, monocrystalline solar cells average between 15-25% efficiency or even higher, while polycrystalline cells have a more modest 13-16% efficiency rate.
Under lab conditions, some thin-film solar cells are able to produce up to 30% efficiency, according to a 2019 research published in Nature Communications. The study, called “Gallium arsenide solar cells grown at rates exceeding 300 µm h−1 by hydride vapor phase epitaxy”, was produced by Wondwosen Metaferia, Kevin L. Schulte, John Simon, Steve Johnston, and Aaron J. Ptak. It showed the use of dynamic hydride vapor phase epitaxy (D-HVPE) to achieve very high growth rates for gallium arsenide (GaAs) and gallium indium phosphide (GaInP) materials. This is significant because higher growth rates carry the potential to lead to lower manufacturing costs for III-V materials, which are typically expensive to produce and are used in specialty applications like space power and high-concentration systems.
It’s important to note that while this efficiency of nearly 30% is significant, almost approaching the theoretical limit for solar panels (called the Shockley-Queisser limit), it is not possible to produce these results in real-world applications. This is because concentrated illumination is used within lab conditions to produce results that are not otherwise achievable under normal light exposure.
This difference in efficiency between film-thin solar cells and other types is mainly due to the structural and material characteristics of the cells. Monocrystalline cells, made from a single, pure silicon crystal, offer a more direct path for electrons, thereby enhancing efficiency. Polycrystalline cells, though slightly less efficient than monocrystalline, still benefit from being composed of multiple silicon crystals.
Thin-film cells, on the other hand, with their characteristically thin photovoltaic layers, have fewer materials to absorb sunlight and generate electrons. The reduced thickness of the photovoltaic layers makes thin-film solar cells more flexible, but this also results in a reduced capacity to efficiently absorb sunlight and generate electricity.
How Flexible Are Thin-Film Solar Cells?
Thin-film solar cells are extremely flexible, and this flexibility sets them apart from traditional crystalline silicon cells. The flexibility of film-thin solar cells stems from their construction: thin layers of photovoltaic materials are applied onto a flexible substrate, such as plastic or metal. Unlike the thicker, rigid silicon wafers in standard solar panels, these thin layers bend and conform to different shapes without breaking.
The adaptability of thin-film-solar cells enables them to be molded into a variety of shapes and sizes, tailored to specific applications. For instance, they are integrated into the curved surfaces of certain building materials or used in portable solar chargers that require lightweight and flexible components. Additionally, thin-film dollar cells are often custom-designed for unique uses such as solar-powered backpacks or transport vehicles where standard rigid panels would be impractical.
What are the types of Thin-film Solar Cells?
There are several types of thin-film solar cells, each with distinct materials, advantages, and applications. The four main types of thin-film solar cells are listed below.
- Amorphous Silicon (a-Si) Solar Cells: a-Si cells use non-crystalline silicon, allowing them to be flexible and bend to different shapes. They are cost-effective, using less silicon and cheap materials for substrates. A drawback is their lower efficiency, requiring larger arrays for the same power.
- Cadmium Telluride (CdTe) Solar Cells: CdTe solar cells are easy to manufacture and absorb sunlight well, particularly at shorter wavelengths. However, they contain toxic cadmium, posing health risks during manufacturing and disposal. Their efficiency typically falls between 9% and 11%.
- Copper Indium Gallium Selenide (CIGS) Solar Cells: CIGS cells have an environmentally friendly manufacturing process and perform well in diffuse light. They are less efficient in humid environments and exhibit efficiencies between 12% and 15%.
- Gallium Arsenide (GaAs) Solar Cells: GaAs cells have high-temperature resistance, making them suitable for environments like the automotive industry. However, they are expensive to produce and prone to higher rates of degradation, despite having efficiencies nearing 30%.
1. Amorphous Silicon (a-Si) Solar Cells
Amorphous Silicon Solar Cells (a-Si) are distinguished by their use of non-crystalline silicon, which is silicon in a form that lacks a definite crystalline structure. This distinct atomic arrangement means that the silicon atoms are not organized in a homogeneous, regular pattern as they are in crystalline silicon. The lack of structure of amorphous cells is what allows them to be more flexible, as the material is applied in very thin layers that bend and conform to different shapes without compromising their structural integrity. The atomic structure of amorphous silicon is particularly effective at absorbing light in low-light conditions.
In terms of advantages, amorphous solar cells are cost-effective to manufacture, requiring only a fraction of the silicon used in other types. A-Si cells utilize readily available and cheap materials like glass, plastic, or stainless steel for their various substrates. Another benefit of amorphous silicon solar cells is their higher tolerance to damage, which means a partially damaged panel does not significantly impact the overall power output.
Amorphous solar cells perform well under various conditions, such as low light or high temperatures. Unlike crystalline cells, amorphous silicon solar cells maintain efficiency better in warmer conditions or when there isn’t as much sunlight available. A-si cells are flexible, making them better suited to certain applications like building-integrated photovoltaics (BIPV) than traditional panels. BIPV solar panels are designed to replace conventional building materials in parts of the building envelope, such as the roof, skylights, or facades.
Recently, scientists in Korea have developed flexible, transparent solar cells using hydrogenated amorphous silicon, showcasing improvements in efficiency and the potential for integration into various surfaces for building-integrated photovoltaics. This work was published in 2023 in the journal “npj Flexible Electronics” by a team including Soo-Won Choi, Jae-Ho Park, Ji-Woo Seo, Chaewon Mun, Yonghun Kim, Pungkeun Song, Myunghun Shin and Jung-Dae Kwon. It demonstrates advancements in thin-film solar technology, highlighting the promise of cost-effective and lightweight solar energy solutions. Another application of amorphous silicon cells is portable solar chargers for electronic devices, given that they are flexible and lightweight compared to traditional solar.
The main drawback of amorphous silicon solar cells is that they are prone to efficiency degradation caused by light exposure over time. This phenomenon, known as the Staebler-Wronski effect, occurs more rapidly than in crystalline silicon cells. In addition, due to their low efficiency, larger arrays of a-Si cells are necessary to generate the same power as smaller arrays of more efficient traditional cells, making them less ideal for limited spaces.
Regarding efficiency, amorphous silicon solar cells reach a maximum efficiency of about 15%. This efficiency level is a result of the inherent properties of amorphous silicon, including its atomic arrangement and light absorption ability. While a limit efficiency of 15% is lower compared to other solar cell types, this disadvantage of amorphous silicon solar cells is offset by their cost-effectiveness and temperature resistance.
2. Cadmium Telluride (CdTe) Solar Cells
Cadmium Telluride (CdTe) solar cells are a type of thin-film photovoltaic technology that uses a layer of cadmium telluride to convert sunlight into electricity. The material in these cells, made from a compound of cadmium and tellurium, is applied in a thin layer onto a substrate typically made of glass or metal.
One of the main advantages of CdTe solar cells is how easy it is to manufacture them. Unlike crystalline silicon cells, CdTe cells use a combination of cadmium sulfide and cadmium telluride molecules. This composition inherently creates the electric field necessary for converting solar energy into electricity, simplifying the manufacturing process compared to the more complex production of silicon solar panels. Another notable benefit of CdTe solar cells is their optimal absorption of sunlight. They are particularly adept at capturing energy at shorter wavelengths, especially more effectively than silicon panels. The efficiency of CdTe solar cells in light absorption is due to the material's ability to absorb sunlight near the ideal wavelength for solar energy conversion.
However, the use of cadmium in CdTe solar cells presents specific health concerns, predominantly during the manufacturing and disposal stages. Cadmium, a highly toxic material, poses significant risks if ingested, inhaled as dust, or handled improperly. In the production of CdTe solar cells, workers face exposure to cadmium, leading to potential lung damage and serious respiratory problems. Additionally, cadmium is a known carcinogen, making prolonged exposure particularly hazardous in industrial settings. For consumers using CdTe solar panels, the risk is substantially lower.
The cadmium is securely encapsulated within the panels, posing negligible risk during regular operation and usage. However, concerns arise at the end of the panel's life cycle. Proper disposal or recycling of these panels is crucial, as damage to the panels might release cadmium. As a result, it is advised that end-of-life CdTe panels are handled by professionals equipped to safely manage their disposal or recycling, thus minimizing health risks to consumers and the environment.
The efficiency of Cadmium Telluride (CdTe) solar panels typically falls between 9% and 11%. This efficiency range is lower when compared to other types of thin-film solar cells like Copper Indium Gallium Selenide (CIGS), which generally exhibit efficiencies between 12% and 15%. While CdTe solar cells offer several manufacturing and cost advantages, their relatively lower efficiency means that they lag behind not only the more traditional crystalline silicon cells but also other thin-film variants.
3. Copper Indium Gallium Selenide (CIGS) Solar Cells
Copper Indium Gallium Selenide (CIGS) solar cells are a category of thin-film photovoltaic technoluses. CIGS uses a combination of copper, indium, gallium, and selenium to convert sunlight into electricity. These components are layered together and applied to a substrate, creating a thin and efficient photovoltaic cell.
One of the key benefits of CIGS solar cells is their environmentally friendly manufacturing process. Producing these cells generates only 12–20g of CO2 equivalent per kWh, a carbon footprint that closely rivals that of wind energy. This makes CIGS an attractive option for sustainable energy initiatives. Additionally, Copper Indium Gallium Selenide cells are particularly effective in diffuse light environments, making them versatile for various geographic locations, including areas with less direct sunlight. Their low-temperature coefficient of -0.36%/ºC also means they suffer less power loss in extreme climates, enhancing their efficiency in a wide range of weather conditions.
In terms of disadvantages, CIGS solar cells have a vulnerability to water vapor, which often leads to sodium migration within the cell and consequently increases degradation. The sensitivity exhibited by CIGS solar cells makes them less suitable for areas with high humidity and intense sunlight, especially if the quality of the product is uncertain.
In terms of efficiency, commercial CIGS modules typically exhibit efficiencies between 12% and 15%. This efficiency range makes them competitive within the thin-film solar cell market, balancing their environmental benefits with a strong performance in energy conversion.
4. Gallium arsenide (GaAs) Solar Cells
Gallium Arsenide (GaAs) solar cells are a specialized type of photovoltaic technology that utilizes the compound gallium arsenide. This material is known for its exceptional semiconductor properties, particularly its efficient conversion of sunlight into electricity and its ability to operate effectively under high temperatures.
One of the primary advantages of GaAs solar cells is their remarkable temperature resistance. Unlike traditional silicon solar cells, which typically lose functionality at around 392°F (200°C), GaAs cells maintain effective functionality at temperatures as high as 482°F (250°C).
The high-temperature resistance exhibited by GaAs solar cells makes them particularly suitable for use in environments like the automotive industry, where cells are exposed to significant heat due to real-time charging, discharging, and energy generation.
The disadvantages of Gallium Arsenide (GaAs) solar cells stem mainly from their complex and costly production process and a tendency towards higher rates of solar degradation. The production process for GaAs solar cells is intricate and expensive for several reasons. First, the raw materials, gallium, and arsenic, are less abundant and more costly than silicon, which is widely available and cheaper. Secondly, the manufacturing process of GaAs cells involves sophisticated techniques and equipment. Gallium arsenide is typically produced using high-purity chemical vapor deposition, a process that requires precise control and cleanroom conditions, adding to the production costs.
These factors combined make GaAs cells significantly more expensive to produce than silicon-based cells, limiting their use to niche applications where their high efficiency and temperature tolerance are indispensable. Regarding solar degradation, GaAs solar cells experience a higher rate of degradation compared to other types. This is partly due to their susceptibility to certain environmental factors, such as UV exposure and thermal cycling. Over time, these factors lead to the breakdown of the cell's materials and a decrease in performance. So, while GaAs cells are highly efficient and perform well under high temperatures, these conditions accelerate degradation processes in the long run, impacting their long-term efficiency and overall lifespan.}
In terms of efficiency, GaAs solar cells are among the most efficient available, with research showing record efficiencies. According to a 2019 study published in Nature Communications, it was shown that GaAs solar cells under lab conditions exhibit efficiencies nearing 30%.
This high-efficiency rate is a significant advantage, especially in applications where maximizing power output from a limited surface area is crucial.
What is the Average Cost of Thin-Film Solar Cells?
Thin-film solar panels are priced between $1 and $1.50 per watt on average, presenting a cost-effective solution in the solar panel market. Of the thin-film solar cell types, CdTe cells are the least expensive due to their low manufacturing costs. Some reports on CdTe show prices as low as $0.46/Watt. Amorphous silicon solar cells cost between $0.50 to $1.50 per watt, while CIGS is in a range of $0.60 – $0.70. GaAs are more costly, with their price being around $50 per watt. The cost of a portable thin-film station, which is a common application of thin-film solar technology, ranges from under $1,000 to around $5,000. The exact costs, however, depend on the wattage and battery capacity of the system.
What Are the Advantages of Using Thin-Film Solar Cells?
While thin-film solar cells don’t usually reach the efficiency levels of monocrystalline and polycrystalline panels, they come with distinct benefits. The most distinct advantage of thin-film solar cells is their light weight, flexibility, low manufacturing costs, lower carbon footprint, ease of installation and portability, enhanced tolerance to high temperatures, and improved performance in shaded conditions:
Below is more information on each of the seven main advantages of thin-film solar cells.
- Light Weight: Thin-film solar cells are exceptionally lightweight due to their thin layers of photovoltaic material. Traditional silicon cells are typically 200-500 microns (µm) thick, whereas thin-film solar cells typically range from 1-15 µm - thinner than a human hair. This lightweight nature makes them easier to transport, handle, and install, reducing logistical challenges and costs. In 2022, MIT researchers developed paper-thin solar cells that are 1/100th the weight of conventional panels, but able to generate 18 times more power per kilogram. The research, led by M. Saravanapavanantham, J. Mwaura, and V. Bulović, was published in a study titled “Printed Organic Photovoltaic Modules on Transferable Ultra-thin Substrates as Additive Power Sources” in the journal Small Methods.
- Flexibility: The physical composition of thin-film solar cells, involving very thin layers of photovoltaic material applied to a flexible substrate, allows them to bend and conform to various surfaces with ease compared to traditional panels. This flexibility enables their application in a wide range of environments, including curved and irregularly shaped surfaces, offering greater versatility than regular silicon crystalline modules.
- Low Manufacturing Costs: Thin-film solar cells are more affordable to produce compared to polycrystalline and monocrystalline modules. The production costs are sometimes up to 50% lower, making these panels particularly appealing for large-scale projects and solar fields.
- Lower Carbon Footprint: The environmental impact of thin-film solar cells is reduced due to their minimal use of silicon. Their manufacturing process is more energy-efficient compared to traditional panels and results in fewer carbon emissions, contributing to a significantly lower overall environmental footprint.
- Ease of Installation and Portability: The slender design and reduced weight of thin-film solar panels make them a prime choice for portable uses and installations in areas where traditional, heavier silicon panels are impractical. This is particularly beneficial for structures with roofing that cannot support the heavy weight and extensive mounting requirements of conventional silicon panels. Examples of their use include lightweight roof structures, temporary setups, and mobile applications like solar-powered vehicles and portable solar chargers.
- Enhanced Heat Tolerance: Thin-film solar panels are less affected by high temperatures than traditional silicon crystalline cells. This is because their materials and structure are more resilient to heat, allowing them to maintain efficiency even in hotter climates. As a result, these panels are ideal for regions with very hot summers, where standard silicon cells suffer performance drops due to heat.
- Shade Resistance: Thin-film solar cells exhibit exceptional performance in shaded conditions or areas where continuous, direct sunlight is not guaranteed. Unlike traditional solar panels, which often experience significant efficiency drops in partial shade, thin-film cells are less susceptible to such reductions. This resistance makes them particularly suitable for residential settings, where shading from trees, buildings, or other obstacles can be a common issue.
How long do Thin-Film Solar Cells Last?
Thin-film solar cells typically have a shorter lifespan than other types of solar panels, lasting between 10 to 20 years. In comparison, monocrystalline panels generally last 35 to 50 years, and polycrystalline panels around 25 to 35 years. Despite their relatively shorter lifespan, thin-film panels offer the advantage of a faster payback period. This means that the savings on electricity costs they provide will cover their initial cost within approximately 8 years, making them a cost-effective option over time.
Are Thin-Film Solar Cells suited for Residential Use?
No, thin-film solar cells are not an ideal choice for residential use, primarily due to their lower efficiency, which ranges from 7-22%. The lower efficiency of thin-film solar cells means they are not as good at converting sunlight into electricity compared to more efficient types like monocrystalline or polycrystalline solar cells.
The reason for the low efficiency of thin-film solar cells lies in the physical properties of their materials, which do not absorb sunlight as efficiently as the crystalline silicon used in other types of solar panels. Consequently, thin-film solar cells produce less electricity per square foot. These limitations are a significant drawback for residential settings where roof space is often limited, as the number of panels required to produce a sufficient amount of electricity would be much higher with thin-film solar cells.
What are the Differences Between Thin-Film Solar Panels and Other Types of Solar Panels?
The main difference between thin-film solar panels and other types, such as monocrystalline and polycrystalline, lies in their material composition and structure. Thin-film panels are made with layers of photovoltaic material that are only a few microns thick, resulting in a lightweight, flexible panel. This thin and flexible nature is due to their use of significantly less material, making them more adaptable to various surfaces and installations. In addition to this primary distinction, there are other differences in efficiency, cost, installation, and lifespan. Below is a detailed overview of each difference:
In terms of efficiency, thin-film solar cells generally have lower efficiency and power capacity compared to monocrystalline and polycrystalline types. Their efficiency ranges from about 7% to 22%, while monocrystalline cells average 15-25% and polycrystalline cells 13-16%. Despite this, thin-film panels are more efficient in low-light conditions, such as during sunrise, sunset, or on overcast days, due to their ability to absorb a wide spectrum of light, including ultraviolet and infrared.
Price-wise, thin-film solar panels are typically less expensive than other types. The price per watt is between $1 and $1.50 per watt, while a portable thin-film system ranges from just under $1,000 to about $5,000. In contrast, a comparable crystalline solar power system costs around $2.85 per watt, with a 6kW system typically priced between $5,500 and $9,000. What’s more, thin-film panels offer savings in the long term and often have shorter payback periods than traditional crystalline panels.
Another big difference between thin-film solar panels and other types has to do with the installation process. Installation of both thin-film and crystalline silicon solar panels involves hardware, mounting brackets, an inverter, and connections between the inverter and panels. However, installing thin-film panels is typically easier and faster due to their lighter weight, flexibility, and simpler handling requirements, necessitating fewer mounting brackets and less labor.
Regarding lifespan, thin-film solar panels usually last between 10-20 years, while crystalline silicon panels often last up to 50 years for monocrystalline and up to 35 years for polycrystalline. However, it should be noted that proper installation and maintenance are crucial for maximizing the lifespan of all types of solar panels.